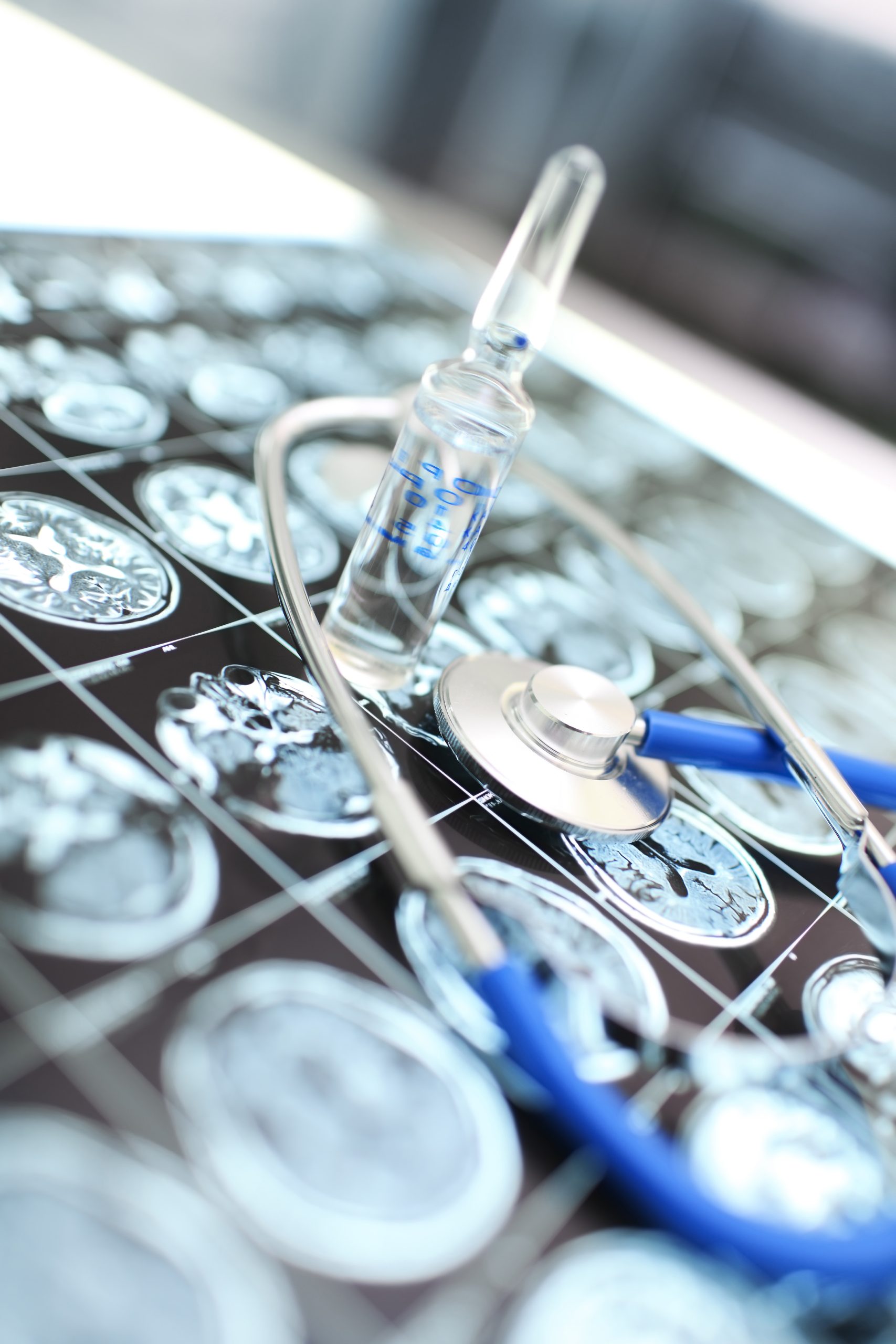
At its core, all research begins with a question. What makes you sick? What’s inside a cell? How does a neuron work? What happens in your body that causes a disease like MS to attack your nervous system?
Building from the basics to the most complex, questions like these drive medical advancements and continually change our understanding of MS. At the cutting edge of new treatment today, we rely on the simplest questions asked many decades ago by scientists and doctors who first began to investigate this disease. Building a body of knowledge is a long and complicated process, with everything working toward where we are today and where we’re headed in the future.
Medical research can be broken down into three main parts: basic science, translational, and clinical. Although the boundaries between the three can sometimes be a little blurry, because of how intricately related they are, it’s a useful framework for us to use in digging deeper into these components.
Basic Science Research
Basic science research in the field of medicine is looking at the inner workings of the human body at the smallest scale – cells, genes, proteins, molecules, and the fundamental processes of biology. It’s what we most often associate with the classic image of scientists in white coats, working in laboratories, peering into microscopes, carefully adding drops of liquid to a petri dish.
Basic science starts out by asking a question or stating a hypothesis, and from there, experiments and tests are designed to get an answer.
“We learn nothing without basic science research,” says Dr. John Corboy, Medical Director of the Rocky Mountain MS Center. “If you believe in the scientific process — that is, an observation is made, a hypothesis is suggested, and you design a study to answer that question — you get whatever the answer to the question is, and then you reframe the next hypothesis around that.”
Ultimately you use the scientific process to try and answer a question that is relevant to the condition that you’re talking about… Then you move the ball towards the goalpost.
— Dr. John Corboy
At its core, basic science research lays the foundation for all medical advancements. One hypothesis and the experimenting and testing conducted around it leads to the next hypothesis, followed by more experiments and tests, which lead to new hypotheses, and so on. In a perfect world, the simplest questions begin a path that progresses toward understanding the most complex questions over time.
“Ultimately you use the scientific process to try and answer a question that is relevant to the condition that you’re talking about, in this case multiple sclerosis, or all the other disorders that we care for,” says Corboy. “Then you move the ball towards the goalpost, and that goalpost is ending the suffering that’s associated with this condition.”
Translational Research
Translational or pre-clinical research aims to take what’s been learned in basic science and begin to apply it to practical use. This starts with developing model interventions, through which scientists and doctors can begin to see what impact they can potentially have on a disease.
It’s not practical or safe to move directly from basic science research to developing treatments for use in patients. Translational research is the critical phase where the potential for treatment approaches is explored.
Testing in the translational research phase often involves cell or animal models of disease, or samples of human or animal tissues. Taking what was learned in basic science, hypotheses here begin to go beyond simple understanding of biology and into questioning what we can do to identify, diagnose, treat, or even cure a disease.
“The basic science really helps us develop incredibly concrete hypotheses that can be tested in translational phases,” said Corboy. “And you can do it in multiple different models that are not necessarily possible in humans.”
Translational research is also commonly where practical diagnostic tests are developed. In MS, a good example of this is the common lumbar puncture used to help many initial diagnoses of MS. Basic science research identified antibodies called oligoclonal bands that were present in the spinal fluid of MS patients. Taking that knowledge, tests were developed to detect these proteins in a patient’s cerebrospinal fluid. If you had a lumbar puncture during your MS diagnosis process, this is exactly what your neurology team was looking for.
A promising new development that further illustrates the importance and promise of this phase of research is Dr. Jeffrey Bennett and Dr. Greg Owens’ work on the identification of an antibody related to MS that could one day fundamentally change diagnosis and possibly even treatment of MS. For more information, please see “Identifying the Target of Antibodies in MS: The Process of Defining a Key Piece of the Puzzle.”
Clinical Research
In medicine, the culmination of the scientific investigation process is in the realm of clinical research. This is where knowledge and discoveries are put into practice, where the long, hard work done in the earlier stages of research are brought to actual human patients.
However, many years of gathering evidence and understanding leading to clinical research doesn’t mean the process speeds up – clinical research, especially the testing of new drugs, is highly-regulated, painstakingly undertaken, and carefully and deliberately monitored to ensure not only accurate and repeatable results, but the safety of the people involved.
Clinical trials are probably the most visible component of clinical research. This is where new drugs and medical interventions are tested on volunteers who’ve chosen to assist researchers in their efforts, and they’re a critical part of bringing any new drug to the general public.
In the United States, clinical trials are regulated by the Food and Drug Administration (FDA), and follow strict guidelines, approval processes, and a basic format that aims to ensure safety and oversight at every step of the process.
The Complex Process of Clinical Trials
The first step in crafting a clinical trial for any new drug is, much like the earlier stages of research, to define a specific question and a plan for getting to the answer. For a new drug, it may be as simple as deciding what the intended outcome of the drug is, and then asking if this drug meets that aim. For existing drugs that have been approved for other uses, testing for a new purpose may mean asking if this drug will work the way researchers expect it to in a different population or on a different disease. This can include information from animal studies, data on toxicity (side effects that cause great harm), manufacturing information, data from any prior human research that might be related to the new drug, and information about the investigator, institution or company pursuing the trial.
Before proceeding, a detailed Clinical Protocol – an outline of the study plan — must also be accepted by the FDA. This protocol includes the following details:
- Who qualifies to participate (include/exclude criteria)
- How many people will be part of the study
- How long the study will last
- Whether there will be a control group and other ways to limit research bias
- How the drug will be given to patients and at what dosage
- What assessments will be conducted, when, and what data will be collected
- How the data will be reviewed and analyzed
Once a study is approved and work begins, researchers start progressing through the “phases” of clinical trials that we commonly hear about in medicine. These phases follow a deliberate and careful process forward, and failures at any stage can pause or even end a study completely, depending on the severity of a failure or if a negative result becomes obvious at an early stage.
The FDA defines the phases of clinical trials as follows:
Phase 1
- Study Participants: 20 to 100 healthy volunteers or people with the disease/condition; For drugs, may involve multiple drug doses
- Length of Study: Several months
- Purpose: To identify safety concerns and refine dosage
- Results: Approximately 70% of drugs continue to the next phase
Phase 2
- Study Participants: Up to several hundred people with the disease/condition
- Length of Study: Several months to two years
- Purpose: To gather data on efficacy of the treatment and identify side effects
- Results: Approximately 33% of drugs continue to the next phase
Phase 3
- Study Participants: 300 to 3,000 volunteers with the disease/condition
- Length of Study: One to four years
- Purpose: Continue gathering efficacy data and monitor for adverse reactions
- Results: Approximately 25-30% of drugs continue to the next phase; If successful and the drug or device is acceptably safe, the drug may be then approved by the FDA for the purpose and population in the Phase 3 trials (typically two Phase 3 trials required)
Phase 4
- Study Participants: Several thousand volunteers with the disease/condition
- Length of Study: Several months to many years
- Purpose: Large-scale monitoring and gathering data on safety and efficacy
At any point in the process outlined above, the FDA can issue a hold to delay or stop the trial if it has any concerns. But more often than a hold, the FDA will provide comments to the investigators in the hopes that they can improve the quality or safety of the trial. If the FDA is satisfied the investigators have done so, the trial can continue as planned.
The process ends when investigators either decide to end clinical trials, or when they file a marketing application. A marketing application is an indicator that investigators are satisfied that the results of at least two large-scale, controlled clinical trials have been a success, and represents the final stage before a manufacturer can begin selling a drug to the public.
“Basic science, biology, chemistry, biochemistry, cellular biology, genetics… none of the clinical trials would mean anything without having all of the basic science first and then the translational research second,” said Corboy. “So for us, we sort of think of them all melting into one another. But I think a lot of times people focus on the sort of the end product, if you will, which makes sense.”
Asking Questions, Learning from Answers
Ultimately, the spectrum of research is focused on asking the right set of questions, learning from the answers, and using that understanding to ask the next right set of questions. Often, the answers aren’t exactly what researchers hope… but even negative answers play an important role in science.
“A big part of it is understanding the process,” says Corboy. “The process will have things that work, and will have things that don’t work. You don’t go in with a bias, you go in with the hypothesis and then you try and come up with something that actually does work.”
Negative research results may look like failures, but in reality we’re learning more and more about MS and other diseases with every bit of information uncovered. Negative results can not only protect people from negative consequences, but they also help steer researchers back to more promising avenues.
“The only way you learn is by actually asking the questions,” says Corboy. “With a negative result, you know not go back there, to go down a different pathway.”
“I can list off probably ten or 15 different negative clinical trials that really changed behavior and potentially protected people from something that was dangerous,” said Corboy. “All studies, regardless of the outcome, they’re all part of learning more and more. And once you find out one thing doesn’t work, you know more about why it didn’t work and you can explore other questions or alternatives.”
Limitations of Research
Medical research is a painstakingly slow and often extremely difficult process. Despite decades of research and the development of highly effective medications to treat the disease, science is still lacking a definitive cause for the development of MS.
“We need to have a greater knowledge base as to the pathogenic status of MS,” says Corboy. “That’s what is actually causing the underlying pathology. And so in that context, we can think of five ways that we might want to treat MS.”
Those five potential areas for treatment in MS are:
The Adaptive Immune System – This is our body’s specialized defense system, that learns over time to fight certain infections and develops specific defenses against pathogens we encounter. Adaptive immune system includes T and B cells and lymphocytes, which are the primary targets of many modern MS therapies. This is very important, for example in responses to vaccines, and in MS the acute malfunctions of the immune system attacking the brain and spine as a relapse. All the presently-approved MS DMTs have positive impacts here.
The Innate Immune System – This is our body’s general defense system, or “first line” of defense. It includes your skin, mucous membranes, and non-specialized immune cells that fight general infections and injuries and help “train” the adaptive immune system. In MS, this is very important in progressive MS. Studies are underway to potentially affect microglia, that are particularly relevant in progressive MS.
Remyelination – MS damages the myelin sheathing around nerve cells. Remyelination refers to repairing and regenerating damaged myelin sheaths. No effective treatment is currently available, but early studies have shown at least in principle that this approach may have merit.
Neuroprotection – This refers to the brain’s capacity to protect itself. While there are many neuroprotective measures people can take (healthy diet, exercise, mental and emotional well-being), there is no active treatment intervention to promote neuroprotection in MS. Many medications are also being studied to, for example, help damaged brain and spine cells produce more energy and maintain important cellular functions longer.
Regenerative Therapy – This refers to repairing or regenerating the damage caused by MS. The most challenging of all these approaches, early studies are being done to use neural stem cells to potentially replace damaged cells.
Conclusion
MS has been a well-known and studied disease for over 150 years, and the time it’s taken to understand it better illustrates exactly how complicated research can be. Despite a long history of identification and diagnosis, it’s only relatively recently that we have effective treatments and drug interventions.
Multiple sclerosis was first defined by Jean-Martin Charcot, neurologist at the Hôpital de Salpétrière in Paris in 1868. Yet the first FDA-approved treatment for MS, interferon beta-1b (first sold under the brand name Betaseron in the US and Betaferon elsewhere) wasn’t introduced until 1993.
This delay may seem excessive and certainly frustrating for people living with MS, but determined researchers and doctors over those many years were building the foundation of knowledge needed for today’s advancements. In the 30 years since the introduction of that first MS drug, progress has accelerated and we now have more than 20 FDA-approved treatments, including several highly-effective disease modifying therapies that have proven successful in slowing and even halting MS progression in some patients. In each phase of research, and in testing each new development and drug, we learn more that will continue to help shape new research and developments in the future.
“As much as we know, as much as we learn, there’s still more that we don’t understand,” says Corboy. “And we can’t have the perfect treatment without the perfect understanding of the cause of MS. This is where identification of potential targets for future research, as in the work of Drs. Bennett and Owens is very exciting.”